Rejecting Arsenate; Stem Cell Bodyguards; Immune Cells Make Flexible Choices
Rejecting Arsenate
Not long ago, some unassuming bacteria found themselves at the center of a scientific controversy: a group claimed that these microorganisms, which live in an environment that is rich in the arsenic-based compound arsenate, could take up that arsenate and use it — instead of the phosphate on which all known life on Earth depends. The claim, since disproved, raised another question: How do organisms living with arsenate pick and choose the right substance?
Chemically, arsenate is nearly indistinguishable from phosphate. Prof. Dan Tawfik of the Department of Biological Chemistry says, “Phosphate forms highly stable bonds in DNA and other key biological compounds, while bonds to arsenate are quickly broken. But how does a microorganism surrounded by arsenate distinguish between two molecules that are almost the same size and have identical shapes and ionic properties?”
To investigate, Prof. Tawfik, postdoctoral fellow Dr. Mikael Elias, PhD student Alon Wellner, and lab assistant Korina Goldin, in collaboration with Tobias Erb and Julia Vorholt of ETH Zurich, looked at a protein in bacteria that takes up phosphate. This protein, called PBP (phosphate binding protein), sits near the bacteria’s outer membrane, where it latches onto phosphates and passes them on to pumps that transport them into the cell.
In research that recently appeared in Nature, the team compared the activity of several different PBPs — some from bacteria like E. coli that are sensitive to arsenate, and others, like those from the arsenic-rich environment, which are tolerant of the chemical. While the PBPs in the ordinary bacterium were about 500 times more likely to bind to phosphate over arsenate, that factor jumped to around 5,000 in the arsenic-tolerant bacterium. In other words, to cope with their toxic environment, the bacteria evolved a mechanism of extreme selectivity to ensure their supply of phosphate while keeping the arsenate out.
Dr. Elias then compared phosphate and arsenate binding by crystallizing PBPs along with one of the two compounds. But the initial comparison suggested that when arsenate bound to the protein, it did so in just the same way as phosphate. Dr. Elias suspected that the key might lie in a single, highly unusual bond between a hydrogen atom in the protein and the molecule. This bond had been previously noted but ignored, as phosphate binding occurred with or without it.
To see the difference, the team had to stretch the limits of crystallization technology, getting the resolution to less than one angstrom — fine enough to identify individual hydrogen atoms and compare their bonds. Only then were they able to identify a single disparity: the angles of that unusual hydrogen bond were different. Inside a tight cavity within the PBP structure, phosphate binds at a “textbook angle,” according to Dr. Elias. The slightly larger arsenate molecule, on the other hand, gets pushed up against the hydrogen and bonds at unnatural, distorted angles. Prof. Tawfik thinks that the angle is likely to lead to repulsion between the molecule and other atoms in the cavity, preventing the PBP from passing arsenate into the cell’s interior.
Says Prof. Tawfik, “These findings may go beyond the solving of a biological mystery. Because phosphates are scarce in many environments, there is quite a bit of interest in understanding how this crucial resource is taken up by organisms. This first observation of a PBP discrimination mechanism is an exciting demonstration of the exquisite fine-tuning that enables proteins to distinguish between two nearly-identical molecules.”
Prof. Dan Tawfik’s research is supported by the Adelis Foundation; the estate of Mark Scher; and Ms. Miel de Botton, UK. Prof. Tawfik is the incumbent of the Nella and Leon Benoziyo Professorial Chair.
Stem Cell Bodyguards
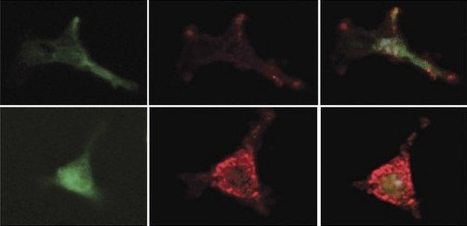
Prostaglandins guard stem cells by increasing the production of an inhibitory factor in the mesenchymal bone marrow cells. Mesenchymal cells are marked with a green protein (left); the inhibitory factor is red (center). Combined image (right): Treatment with prostaglandins (bottom) increases the secretion of the inhibitory factor. From the work of Prof. Tsvee Lapidot
Hiding deep inside the bone marrow are special cells. They wait patiently for the hour of need, at which point these blood-forming stem cells can proliferate and differentiate into billions of mature blood immune cells to help the body cope with infection, for example, or extra red blood cells for low oxygen levels at high altitudes. Even in emergencies, however, the body keeps to a long-term plan: it maintains a reserve of undifferentiated stem cells for future needs and crises. A research team headed by Prof. Tsvee Lapidot of the Weizmann Institute’s Department of Immunology recently discovered a new type of bodyguard that protects stem cells from over-differentiation. In a paper that appeared in Nature Immunology, they revealed how this rare, previously unknown subgroup of activated immune cells keeps the stem cells in the bone marrow “forever young.”
Blood-forming stem cells live in comfort in the bone marrow, surrounded by an entourage of support cells that cater to their needs and direct their development — the mesenchymal cells. But the research team, which included postdoctoral fellow Dr. Aya Ludin, Prof. Steffen Jung of the Department of Immunology and his group, and Ziv Porat of the Biological Services Unit, discovered another type of support cell for the stem cells. These are an offshoot of the macrophage family, literally the “big eaters” of the immune system that are important, for instance, for bacterial clearance. The team found, however, that a rare sub-population of the bone-marrow macrophages has another role to play. Each of these rare macrophages can take a stem cell under its wing and prevent its differentiation.
Probing more deeply, the researchers revealed, in precise detail, how these macrophages guard the stem cells. They secrete substances called prostaglandins, which are absorbed by the stem cells. In a chain of biochemical events, these substances delay differentiation and preserve the youthful state of the stem cells. In addition, the prostaglandins work on the neighboring mesenchymal cells, activating the secretion of a delaying substance in them and increasing the production of receptors for this substance on the stem cells themselves. This activity, says Prof. Lapidot, may help the non-dividing stem cells survive chemotherapy — a known phenomenon. Macrophages also live through the treatment, and they respond by increasing their prostaglandin output, thus heightening their vigilance in protecting the stem cells.
The bodyguard macrophages also increase their activity in times of infection. While other members of the macrophage family are recruited to fight the pathogens, their cousins in the bone marrow are hard at work ensuring that a pool of stem cells will resist the urge to differentiate. In previous work in Prof. Lapidot’s lab, it was discovered that prostaglandin treatments can improve the number and quality of stem cells. Doctors are currently testing this insight in clinical transplantation trials for the use of stem cells from umbilical cord blood to treat adult leukemia patients. These trials are showing that prior treatment with prostaglandins improves migration and repopulation potential, enabling the small quantities of cord-blood stem cells to better cure the patients. “The present study hints at the possibility of further increasing the support for bone marrow stem cells by exploring this intriguing connection between the immune cells and stem cells,” says Prof. Lapidot. “An understanding of the mechanisms at work in these cells might improve the success of stem cell transplantation, especially that of umbilical blood.”
Prof. Steffen Jung’s research is supported by the Leir Charitable Foundations; the Leona M. and Harry B. Helmsley Charitable Trust; the estate of Olga Klein-Astrachan; the estate of Lola Asseof; Lord David Alliance, CBE; and the Adelis Foundation.
Prof. Tsvee Lapidot’s research is supported by the Leona M. and Harry B. Helmsley Charitable Trust. Prof. Lapidot is the incumbent of the Edith Arnoff Stein Professorial Chair in Stem Cell Research.
Immune Cells Make Flexible Choices
Our immune systems must be tremendously complex to respond to the unending assault of viruses, bacteria, and cancerous cells. One of the mechanisms used by the immune system to cope with the huge variety of possible threats is to randomly combine DNA segments for the production of receptors on lymphocytes, a type of white blood cell. The number of possible receptors that can be produced in this way is about one thousand times the number of stars in our galaxy — one followed by 15 zeroes. And yet, the actual array of receptors produced does not conform to this picture of random chance: Some receptors are produced at a higher rate than others. New research at the Weizmann Institute can help explain how the immune system maintains its complexity while giving preference to certain receptors.
The research team headed by Dr. Nir Friedman, including postdoctoral fellows Drs. Wilfred Ndifon and Hilah Gal, together with Prof. Ruth Arnon and Dr. Rina Aharoni, all of the Department of Immunology, looked at the DNA sequences for receptors in immune cells called T lymphocytes. These receptors identify disease agents so that they can be destroyed by the immune system. The genetic sequences encoding these receptors are each composed of three random DNA segments — something like the random lineups in a slot machine. Each of those segments is taken from a different area of the lymphocyte cell genome; each area has a full “menu” of segments to choose from. The assembly of the sequence takes place when the DNA strand folds, bringing a segment from the first area close to those in the second and third areas. The sequence is then cut and pasted together, and the excess bits of DNA in between discarded, thus creating a new and unique genetic sequence for the receptors in each lymphocyte cell.
In a study that appeared recently in the Proceedings of the National Academy of Sciences, the team used a system they developed based on advanced high-throughput sequencing techniques to investigate the genetic sequences of an entire array of lymphocyte receptors in mice. With this “panoramic view,” the researchers were able to assess how widespread each receptor was, and even to suggest a reason for the uneven distribution. It appears that the secret is in the pieces of DNA that eventually get discarded: Both the length of these segments and their flexibility — a function of the protein “packaging” that gives them shape — determine how likely it is that two distant segments will meet.
With this insight, the researchers created a model that can predict the production distribution of receptors based on the distance between segments and the flexibility of the DNA. They then looked at small groups of up to five individuals to see if they could find common lymphocyte receptor sequences among them. Surprisingly, the team discovered that a group of five was more likely to share a common sequence than smaller subgroups. That may seem like saying there is a higher chance of winning at the slot machine five times in a row than twice, but the scientists can explain this finding based on the preferences revealed. The common sequences may simply be situated in the genome in such a way that they are more likely to be integrated into the receptor sequences. Such sequences may have been selected by evolution for their ability to fight common disease agents or prevent autoimmune disease.
Says Dr. Friedman: “While our immune system often seems to rely on ‘luck’ to produce random receptors against a long list of threats, the shared receptors suggest that this mechanism is finely tuned to ensure a response to common diseases.”
Prof. Ruth Arnon’s research is supported by the Leona M. and Harry B. Helmsley Charitable Trust.
Dr. Nir Friedman’s research is supported by the Abraham and Sonia Rochlin Foundation; the Clore Center for Biological Physics; the Jeanne and Joseph Nissim Foundation for Life Sciences Research; the Nella and Leon Benoziyo Center for Neurological Diseases; the Crown Endowment Fund for Immunological Research; the Norman E. Alexander Family Foundation; the Carolito Stiftung; the Adelis Foundation; the Victor Pastor Fund for Cellular Disease Research; the estate of Susie Knoll; and the estate of John Hunter. Dr. Friedman is the incumbent of the Pauline Recanati Career Development Chair.