How Water Could Have Flowed on Mars; Pumping Zinc; Microbes Take their Sulfur Light
How Water Could Have Flowed on Mars
A new model suggests volcanic activity in Mars’ distant past spewed enough greenhouse gases to melt ice and warm the atmosphere
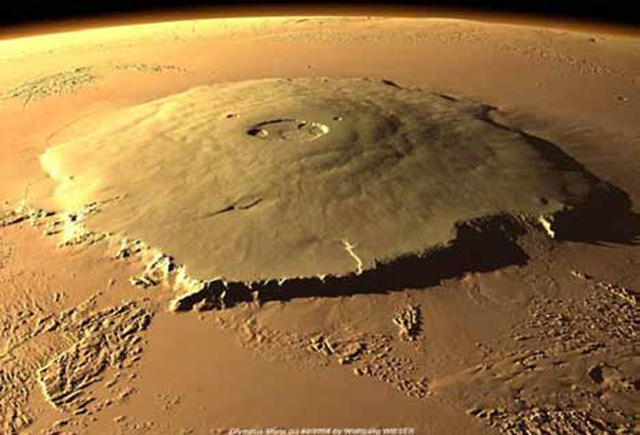
Satellite image of Olympus Mons on Mars, the largest volcano in the solar system at about three times the height of Mount Everest. Around 3.5 to 4 billion years ago, the release of volcanic gases, especially the greenhouse gas sulfur dioxide, may have warmed the surface of Mars episodically, melting the ice and thereby explaining the presence of geomorphological features indicative of the flow of water on the planet’s ancient surface.
Why does the cold, barren surface of Mars contain geological features that appear to have been formed by flowing water: river valleys, lake basins, and deltas? A new model, published online in Nature Geoscience, suggests that sulfur spewed into the Martian atmosphere by ancient volcanoes could have periodically warmed the surface enough for the ice to melt and water to flow.
Indeed, the signs of flowing water have been a puzzle, as the latest generation of climate models portrays Mars as an eternally ice-cold planet with all of its water frozen solid, especially early in its history, when the Sun was weaker than it is today. Today, most of that water is locked in polar caps. Dr. Itay Halevy of the Weizmann Institute of Science’s Department of Earth and Planetary Sciences and Dr. James Head III of Brown University thought the answer might lie in the now dormant volcanoes on the planet’s surface, which could have played a larger role than previously thought in shaping its climate.
On Earth, volcanic emissions – sulfur compounds and ash – tend to cool the climate. But in the presumably dusty early atmosphere of Mars, the net effects might have been different. To understand their impact, Drs. Halevy and Head first calculated the size of ancient volcanic eruptions, based on the volcanic rock formations observed on the planetary surface today. Their estimations show that the eruptions were violent – hundreds of times the force of the average eruption on Earth – and may have lasted up to a decade. This means that the amounts of gases spewed from the mouths of these volcanoes, from what we know of Earth’s eruptions, must have been enormous.
The team’s simulations showed large amounts of the greenhouse gas sulfur dioxide mixing into the atmosphere. But warming caused by the sulfur dioxide was thought to be outweighed by cooling due to the creation of sun-blocking sulfuric acid particles, which form as sulfur dioxide reacts in the atmosphere. Drs. Halevy and Head showed that, in an atmosphere already as dusty as that of Mars, the sulfuric acid mostly forms thin coatings around particles of mineral dust and volcanic ash, subduing the added cooling. The net effect, according to the model the scientists created, was modest warming – just enough to allow water to flow at low latitudes on either side of the planet’s equator.
Liquid water may have flowed in these regions for tens to hundreds of years during and immediately after volcanic eruptions. The model suggests that during these brief, but intense, wet periods, the surface of the planet could have been carved by flowing rivers and streams.
Dr. Itay Halevy’s research is supported by the Sir Charles Clore Research Prize; the Carolito Stiftung; the estate of Olga Klein Astrachan; and the European Research Council.
For the scientific paper, click here.
Pumping Zinc
Weizmann Institute researchers reveal an unusual cellular mechanism that may be faulty in Alzheimer’s disease
Why would a cell go to the trouble of destroying perfectly good, newly minted proteins? New research from the Weizmann Institute and research centers in Germany, recently published in Molecular Cell, suggests that this unusual cellular mechanism may, among other things, be faulty in Alzheimer’s disease.
Dr. Maya Schuldiner and research student Dr. Shai Fuchs of the Weizmann Institute’s Department of Molecular Genetics, working in collaboration with Drs. Marius Lemberg and Donem Avci of Heidelberg University, studied presenilin, a human protein mutated in a familial form of early-onset Alzheimer’s.
To investigate the mechanism of this protein, the researchers looked to the ancestor of presenilin, a yeast protein they identified as Ypf1 (for yeast presenilin fold1), which has been well-conserved throughout evolution. When they removed Ypf1 from the yeast cells, the result was excess quantities of a protein whose role is to pump zinc – an essential metal – into the cell. The puzzling thing was that, although there are two proteins for pumping zinc into the cell, only one was affected. The one increased is a “turbo,” or high affinity, pump; the second, unaffected protein is more of a “workaday,” low affinity pump. Like presenilin, Ypf1 is a protease – a protein that degrades other proteins – so the researchers concluded that its function is to rid the cell of the “turbo” zinc transporters.
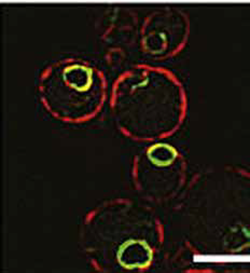
Fluorescent image of Baker’s yeast cells showing that the presenilin-like protein Ypf1 (green) is found in the same cellular area as presenilin: around the nucleus.
In fact, they realized they were looking at a sort of two-pump system, which had first been described several years ago by the Weizmann Institute’s Prof. Naama Barkai. The low-affinity nutrient pumps may not be as efficient at bringing nutrients into the cell, but they are very sensitive to changes in those nutrients; when levels drop, they enable the activation of a backup plan. High-affinity transporters can go into action to stockpile the nutrient in preparation for coming starvation, but these pumps can’t enable the backup plan. In this scenario, healthy cells should work most of the time on the workaday pumps, only allowing the turbo pumps to reach their outer surfaces in times of need.
The researchers asked how excess turbo pumping on the cell’s surface would affect its ability to prepare for scarcity. Indeed, Ypf1-deficient cells were very slow to sense the nutrient’s lack, so they performed poorly during zinc starvation and took longer to recover. And more than zinc pumps are affected: the research showed that in the absence of Ypf1, high-affinity transporters for many other nutrients are deregulated.
“Continually producing high-affinity transporters and then degrading them is a sort of double-safe mechanism that cells evolved to ensure that levels of vital nutrients like zinc remain as stable as possible within the cell,” says Dr. Fuchs. “Though we still don’t know exactly how the mechanism in humans is tied to Alzheimer’s, there is some interesting evidence that zinc transport in particular, and metal transport in general, could play a pivotal role in disease onset and progression.” “We are excited that this new clue may open up fresh directions for thinking about the causes of Alzheimer’s disease, as these are still not well understood,” says Dr. Schuldiner.
Dr. Maya Schuldiner’s research is supported by the Foundation Adelis; the Georges Lustgarten Cancer Research Fund; the Dora Yoachimowicz Endowed Fund for Research; the Berlin Family Foundation; Roberto and Renata Ruhman, Brazil; and Karen Siem, UK.
For the scientific paper, click here.
Microbes Take their Sulfur Light
A model explaining the preferences of deep-sea microbes can help reveal the ancient past – as well as predict the future
On the ocean floor, where oxygen is in short supply, live microbes that “breathe” sulfur instead of oxygen. Far from the world’s forests, these microbes play a vital role in the planet’s carbon cycle, digesting around half of the organic matter that sinks to the seabed. New research, recently published as a feature article in the Proceedings of the National Academy of Sciences (PNAS), combines biochemical principles and stable isotope theory to create a new approach to understanding what the metabolic activity of these common microorganisms tells us about their environment. This approach may prove to be a valuable tool for helping decipher crucial processes in the planet’s ecology – in the past, as well as the present and future.
Dr. Itay Halevy of the Weizmann Institute’s Department of Earth and Planetary Sciences and Dr. Boswell Wing of McGill University sought to explain phenomena that had been observed in experiments for almost 60 years: so-called sulfur-reducing microbes discriminate between the four stable isotopes of sulfur in ways that depend on the microbes’ growth conditions. Through respiration the microbes take in a sulfur compound – sulfate – from their environment and use it to fuel their metabolic reactions. But the microbes prefer using sulfate with the lighter isotopes of sulfur, so they “fractionate” these isotopes, generating metabolic products that are enriched in 32S and depleted in 34S. The experiments reveal that fractionation drops off as respiration rates speed up, and increases as sulfate concentrations increase. These patterns have been used to interpret the geologic record of sulfur isotopes and, thus, past environmental conditions, but a comprehensive model that can explain and predict the patterns has been lacking.
To create their model, the researchers had to incorporate information about biochemical reactions, the various environmental factors that regulate metabolic processes in sulfate-reducing microorganisms, and the chemistry of sulfur isotopes, which are taken up in one chemical form and expelled in another (much like inhaling O2 and exhaling it in the form of CO2). Among other things, says Dr. Halevy, he and Dr. Wing made use of principles – developed in part Prof. Ron Milo’s group in the Department of Plant and Environmental Sciences – that tie biological reaction rates to the energetics of the reactions and the dynamics of the enzymes involved in those reactions.
The ability to model the processes that control microbial isotope fractionation has many possible implications. If the testing that is now underway supports the model, it will be an invaluable tool for understanding microbial activity in present-day, as well as ancient, environments. The researchers suggest that it would enable geoscientists to decode ancient history by understanding how sulfur-reducing microbes left their mark long ago in sulfur-bearing rock formations. Moreover, Dr. Halevy points out, the approach may open doors to other areas of research: “The method can be applied to the microbial metabolism of many additional elements,” he says. “For example, it could be used to model the nitrogen isotope fractionation of the denitrifying bacteria that drive an important part of the planet’s nitrogen cycle, or that of the microorganisms which produce the greenhouse gas methane.”
Dr. Itay Halevy’s research is supported by the Sir Charles Clore Research Prize; the Carolito Stiftung; the estate of Olga Klein Astrachan; and the European Research Council. Dr. Boswell Wing worked in the lab of Dr. Itay Halevy through the Feinberg Foundation Visiting Faculty Program.
For the scientific paper, click here.